An Introduction to Capillary Electrophoresis: Theory, Practice and Applications
How does capillary electrophoresis work and why might you choose it over liquid chromatography?
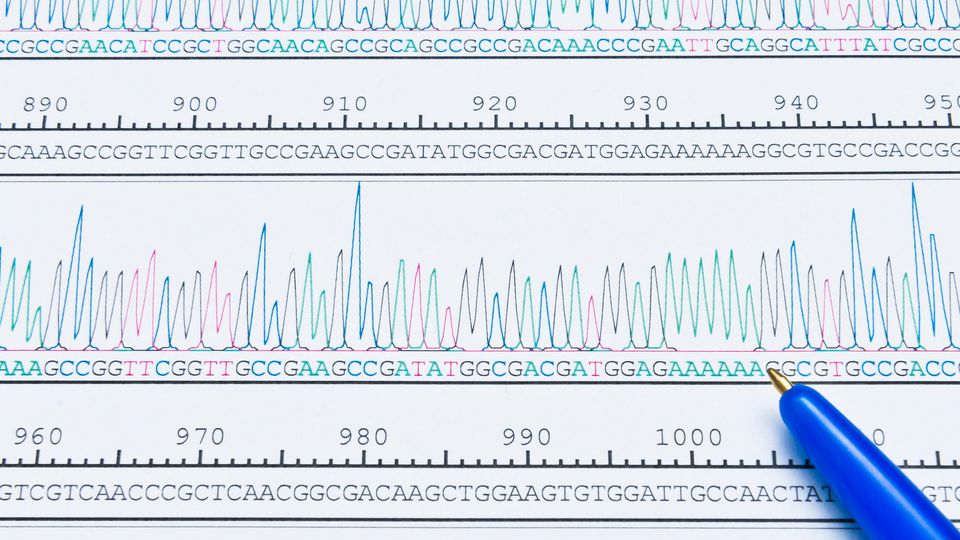
Complete the form below to unlock access to ALL audio articles.
Capillary electrophoresis (CE) is the name of a family of analytical techniques that involve the separation of charged molecules within a narrow capillary tube under the influence of an electric field.
What is capillary electrophoresis?
How capillary electrophoresis works, ionic mobility and the capillary electrophoresis instrument
Types of capillary electroseparation, their strengths and limitations
- Capillary zone electrophoresis (CZE)
- Capillary gel electrophoresis (CGE)
- Capillary isoelectric focusing (CIEF)
- Capillary isotachophoresis (CITP)
- Micellar electrokinetic capillary chromatography (MEKC)
- Capillary electrochromatography (CEC)
How does capillary electrophoresis compare to liquid chromatography?
Capillary electrophoresis applications
The origins of these techniques (Figure 1) can be traced to the early development of electrophoresis in the 1930s by the Swedish biochemist Arne Tiselius.1 In the 1950s, scientists, including the Swedish biochemist Stellan Hjertén, introduced the use of chromatographic capillary tubes to the electrophoretic techniques in response to a need to use smaller amounts of sample and improve separation efficiency.2 In the 1980s, James W. Jorgenson and Krynn D. Lukacs introduced the use of fused silica capillaries of less of 100 μm, significantly improving the performance of the technique, which lead to its popularization.3 Today, CE is a powerful separation and analysis technique that offers quick and efficient results, performing better in many cases than other techniques such as liquid chromatography (LC). For this reason, CE is widely employed in pharmaceutical and biotechnological industries.4
Figure 1: Historical milestones of CE development. Credit: The Author.
What is capillary electrophoresis?
In general, electrophoresis techniques are based on the premise that different charged chemical species in a sample move at different velocities when an electric field is applied. These distinct mobilities, which depend on the charge and size of the molecules, enables the separation of the analytes present in a sample.5, 6, 7 Although all electrophoresis techniques share these fundamentals, there are many methodologies that can be classified into four groups (Figure 2):8
Figure 2: Classification of electrophoretic techniques. Credit: The Author.
- Gel electrophoresis: separation takes place through a gel matrix.
- Zone electrophoresis: molecules are separated into zones as a function of their size.
- Free flow electrophoresis: separation occurs through a buffer flow between two glass plates.
- Capillary electrophoresis: analytes separate within a fused silica capillary.
What sets CE apart from other electrophoretic techniques is its utilization of a fused silica capillary as the separation medium. This microscale system offers numerous advantages, including high separation efficiency, rapid analysis, minimal sample consumption and compatibility with various detection methods such as UV-absorbance, fluorescence and mass spectrometry. Furthermore, CE can be performed in various modes (which will be discussed later), each tailored to specific separation requirements. Unlike the widespread gel electrophoresis, CE does not require extensive gel preparation or offer limited separation lengths. Instead, it relies on the electroosmotic flow phenomenon, wherein a controlled flow of buffer solution moves the analytes through the capillary, enhancing separation efficiency. In contrast to other electrophoretic techniques, CE offers superior resolution due to its ability to mitigate diffusion effects and enhance reproducibility. The small capillary dimensions and precise control of experimental parameters grant CE a distinctive edge over conventional methods. While gel electrophoresis depends on the migration of molecules through a porous gel matrix, and other electrophoretic methods may involve larger separation platforms, CE stands out for its high-resolution separation on a microscale. 5, 6, 7
How capillary electrophoresis works, ionic mobility and the capillary electrophoresis instrument
As explained before, electrophoretic separation is possible thanks to the different velocity at which chemical species move when an electric field is applied. This velocity is proportional to a physical magnitude known as ionic or electrophoretic mobility, μep, which depends on the charge (q) and size (ri) of the molecule in the following manner:
With η being the viscosity of the medium, this expression shows that the electrophoretic mobility is proportional to the charge/size ratio (q/ri) and that the displacement direction is determined by the sign of the charge (Eq. 1). 5, 7
μep = q Eq. 1
6πηri
In CE, the mobility of ions is affected by an additional phenomenon called “electroosmotic flow” (EOF). Fused silica capillaries are the type most commonly employed in CE and their inner surface displays ionizable moieties that present negative charge at pH values above 4. As a consequence, positive ions from the passing buffer solution become attached to the inner surface of the capillary, creating a very static layer (called a Stern layer). Next to the Stern layer, a more diffuse layer of ions is created (known as the outer Helmholtz layer) (Figure 3A). When an electrical field is applied, positive ions from the outer Helmholtz layer move to the cathode, dragging associated solvent molecules, which results in the generation of an EOF inside the capillary. This flow displays a uniform front, in contrast to the parabolic front of the more common hydrodynamic flow (Figure 3B), which results in a highly enhanced efficiency of the separation compared to other techniques. 5, 7
Figure 3: A: Scheme of the ion distribution at the inner surface of a fused silica capillary. B: Comparison of the front profile of the flow for both electroosmotic and hydrodynamic flows. Credit: Technology Networks.
Because of the presence of the EOF, the mobility of the ions is redefined as an apparent mobility, μapp:
μapp = μep + μEOF Eq. 2
Subsequently, the separation of the chemical species in CE is determined by the joint action of the electrophoretic mobility and the electroosmotic mobility (Figure 4).5, 7
Figure 4: Scheme of the migration of chemical species occurring in CE and its dependence on charge and size. Credit: Technology Networks.
The equipment for CE is relatively simple. In the case of the most commonly used CE technique, capillary zone electrophoresis (CZE), the capillary is filled with an electrolyte solution, and an electric field is applied across it. The capillary itself consists of three key regions: the injection end, separation region and detection point (Figure 5). At the injection end, a small sample volume is introduced. The separation region, where the actual separation takes place, is typically coated with a polymer to minimize interactions with the capillary wall. As the electric field is applied, positively charged ions (cations) migrate towards the negatively charged cathode, while negatively charged ions (anions) move towards the positively charged anode. Bearing in mind Eq. 1, smaller, highly charged molecules will move faster than larger or less charged ones. Neutral molecules will migrate unseparated to the cathode by the action of the EOF. When the analytes reach the detection point, they are registered by the detector (UV-visible, fluorescence, mass spectrometry…) and then displayed as peaks in a spectrum called an “electropherogram”. In this electropherogram, different peaks corresponding to different analytes are displayed at the time they reach the detector, with the peak area being directly proportional to the amount of analyte in the sample. 5, 6, 7, 9
Figure 5: Schematic representation of a typical CE instrument. Credit: Technology Networks.
Types of capillary electroseparation, their strengths and limitations
As mentioned before, CE encompasses a set of techniques with different characteristics that allow adaptation to diverse separation or sample requirements. The most relevant CE techniques are summarized in the next paragraphs and compared in Table 1.
Capillary zone electrophoresis (CZE)
CZE is the most commonly used CE technique and its basic working principles have been explained in the previous section. CZE is characterized by its minimal sample preparation requirements and speed. This technique is particularly suitable for ions and small molecules, but its resolution might be limited when dealing with complex mixtures, as closely related species can elute in broad peaks.10
Capillary gel electrophoresis (CGE)
CGE enhances separation by incorporating a sieving matrix, typically a cross-linked polymer, in the capillary. This matrix retards the migration of larger molecules more than smaller ones, resulting in high-resolution separations. CGE is particularly well-suited for the separation of nucleic acids and proteins, enabling accurate analysis of molecular weight differences. However, CGE's reliance on the gel matrix makes it slower compared to other techniques.11, 12, 13
Capillary isoelectric focusing (CIEF)
This technique exploits differences in the isoelectric points (pI) of analytes to achieve separation. The capillary is filled with an ampholyte buffer, creating a pH gradient. Analytes migrate to their respective pI, where they become electrically neutral and halt. This technique is excellent for proteins and peptides with distinct pIs. However, CIEF is time-consuming due to the need for pI calibration, and complex samples can challenge resolution.14, 15, 16
Capillary isotachophoresis (CITP)
In CITP, the separation of analytes is based on their ionic mobilities in a stepwise manner. A leading electrolyte with lower mobility and a trailing electrolyte with higher mobility sandwich the analytes, driving them toward the detector. CITP excels in separating complex mixtures, ensuring that each analyte occupies a distinct migration zone. However, concentration effects can lead to distortion of peak shapes.17, 18
Micellar electrokinetic capillary chromatography (MEKC)
MEKC is a hybrid technique that merges electrophoresis and chromatography. It involves adding surfactant micelles to the electrolyte. Neutral analytes partition between the aqueous phase and the micelle core, while charged analytes migrate according to their charge-to-mass ratio. MEKC's versatility allows for the separation of both neutral and charged compounds. However, micelle stability can affect reproducibility.19, 20
Capillary electrochromatography (CEC)
CEC combines chromatography and electrophoresis principles. A stationary phase coats the inner capillary wall, interacting with analytes similarly to traditional chromatography. This interaction, combined with electrophoretic mobility, offers high-resolution separations. CEC is compatible with various detection methods and is capable of separating complex samples. However, the optimization of stationary phases and mobile phases can be intricate.21, 22, 23, 24
Table 1: Summary of electrocapillary separation techniques with their strengths and limitations.
Technique | Strengths | Limitations |
CZE | Rapid, minimal preparation, suitable for ions/small molecules. | Limited resolution for complex mixtures. |
CGE | High resolution, suitable for nucleic acids/proteins. | Slower due to gel matrix. |
CIEF | Separates based on pI, excellent for proteins/peptides. | Time-consuming, challenging with complex samples. |
CITP | Efficient for complex mixtures. | Concentration effects. |
MEKC | Versatile, separates neutral/charged compounds. | Micelle stability affects reproducibility. |
CEC | High resolution, compatible with detectors. | Complex optimization. |
How does capillary electrophoresis compare to liquid chromatography?
CE and LC are powerful separation techniques widely employed in analytical chemistry, each offering distinct principles and applications. Both techniques share some common features: Firstly, they involve the separation of complex mixtures based on the interaction of analytes with a mobile phase and a stationary phase. Then, both techniques can achieve high-resolution separations of a wide range of compounds, including small molecules, ions, proteins and biomolecules. And finally, they are compatible with various detection methods, such as UV-absorbance, fluorescence and mass spectrometry, enabling robust and versatile analyses.5, 7, 25, 26
The fundamental difference between CE and LC lies in the driving force behind separation. Whereas CE relies on the differential migration of charged species under an electric field, LC capitalizes on differential interaction between analytes and a stationary phase within a column. In addition, CE typically offers rapid separations, while LC allows for greater control over separation parameters, making it suitable for complex mixtures.5, 7, 25, 26
Since both CE and LC present advantages and limitations (summarized in Table 2), some criteria must be taken in consideration in order to choose the technique that fits better to the studied sample and the available resources (Table 3).
Table 2: Summary of the advantages and disadvantages of CE and LC.
Technique | Advantages | Disadvantages |
CE | - Minimal sample consumption and waste production. - High separation efficiency due to microscale dimensions and uniform flow front. - Rapid analysis and reduced run times. - Excellent for charged molecules and fast separations. | - Limited capacity for separating large molecules. - May require specialized equipment for certain applications. - Sensitive to changes in buffer composition.
|
LC | - Versatile separation of a broad range of molecules. - Enhanced control over separation conditions. - Suitable for complex samples and biomolecules. - Many mobile and stationary phases are available, as well as columns of different lengths and packings. | - Longer analysis times compared to CE. - Consumes large sample volumes. - Risk of column deterioration and band broadening. - More complex instrumentation.
|
Table 3: Criteria for the selection of CE or LC as separation and analysis technique.
Choose CE over LC | - Analytes are charged or ionic in nature. - Rapid analysis is crucial. - High separation efficiency is required for small molecules. - Minimal sample consumption is required. |
Choose LC over CE | - Analytes vary in size, charge and hydrophobicity. - Superior separation control and flexibility are needed. - Complex samples with a diverse range of compounds are being analyzed. - Longer separations times are acceptable. |
Capillary electrophoresis applications
CE has emerged as a versatile analytical technique standing out because of its ability to separate charged molecules with remarkable precision and speed. For this reason, CE is utilized in diverse fields and applications, such as:
- DNA/RNA analysis: CE plays a pivotal role in genomics, facilitating DNA sequencing, fragment analysis and mutation detection.27, 28 For instance, Sanger sequencing utilizes CE to unravel genetic codes.29 In RNA analysis, CE aids in transcriptomics, revealing gene expression patterns.30, 31 Additionally, CE's rapid separations and high-resolution capabilities enhance genotyping, something crucial for personalized medicine.32, 33
- Food analysis: CE contributes to food safety and quality control. Detection of allergens, additives and contaminants is streamlined by CE's sensitivity.34, 35 It enables the quantification of vitamins, amino acids and fatty acids, ensuring nutritional label accuracy.36 CE also helps to detect food product adulterations.37
- Environmental monitoring: CE stands as an indispensable tool in environmental testing of pollutants, heavy metals and organic compounds in water and soil samples.38, 39, 40 Detection of pesticide residues and pharmaceutical pollutants aids in safeguarding ecosystems.41, 42 CE also assists in monitoring water quality, allowing the quantification of relevant anions like nitrates and sulfates.43, 44
- Pharmaceutical analysis: CE expedites drug development, allowing quantitative determination of drugs to both assure maximum purity and the absence of dangerous impurities, ensuring product safety and efficacy.45, 46 Furthermore, the production of many drugs involves the selection and isolation of enantiomers. In this regard, CE-based chiral separations enable enantiomeric purity assessment, which is crucial since the presence of undesirable enantiomers may result in adverse effects.47, 48
- Diagnostics: CE can be used as a tool for diagnostics through biomarker detection or protein profiling. It is possible to detect and quantify electrolytes, enzymes and hormones in body fluids, aiding disease diagnosis.49, 50, 51 In this context, CE-based immunoassays have been developed offering rapid and precise protein quantification.52, 53
- Proteomics: CE applied to the study of proteins and peptides allows post-translational modifications to be uncovered and protein interactions to be identified.54, 55, 56, 57 This information contributes to the discovery of potential drug targets and disease biomarkers.
- Forensic science: CE facilitates forensic DNA analysis, vital in criminal investigations and paternity testing. The technique's sensitivity enables the analysis of minute samples, often crucial in solving cold cases.58, 59, 60
- Biotechnology: CE is employed by biotechnological companies to ensure the quality of products such as recombinant proteins or monoclonal antibodies. CE-based assays assess protein folding and glycosylation, pivotal for biotherapeutic efficacy and safety.61, 62, 63
CE has become one of the most versatile techniques to perform analysis of samples of a wide variety of molecules, from metal ions to organic compounds or biomolecules. Due to its resolution, speed and efficiency, it surpasses other conventional techniques such as LC in many aspects, and has become a routine technique in many diverse fields.
1. Tiselius A. A new apparatus for electrophoretic analysis of colloidal mixtures. Trans Faraday Soc. 1937; 33:524-531. doi:10.1039/TF9373300524
2. Hjerte’n S. Stellan Hjerte’n, Uppsala University. Analyst. 2003; 128(11): 1307-1309. doi:10.1039/B307798P
3. Jorgenson JW and Lukacs KD. Free-zone electrophoresis in glass capillaries. Clin Chem. 1981; 27(9): 1551-1553. doi:10.1093/clinchem/27.9.1551
4. Sastre Toraño J, Ramautar R and de Jong G. Advances in capillary electrophoresis for the life sciences. J Chromatogr B. 2019; 1118-1119: 116-136. doi:10.1016/j.jchromb.2019.04.020
5. Shallan A, Guijt R and Breadmore M. Capillary electrophoresis: Basic principles. In: Siegel JA, Saukko PJ and Houck MM, ed. Encyclopedia of Forensic Sciences. Second edition. Academic Press; 2013:549-559. doi:10.1016/B978-0-12-382165-2.00241-5
6. Harstad RK, Johnson AC, Weisenberger MM and Bowser MT. Capillary electrophoresis. Anal Chem. 2016; 88(1):299-319. doi:10.1021/acs.analchem.5b04125
7. Chapter 1. Schmitt-Kopplin P and Fekete A. The CE-way of thinking: “All is relative!”. Schmitt-Kopplin P, ed. Capillary Electrophoresis. Methods and Protocols. Second edition. Humana Press; 2016:3-19. doi:10.1007/978-1-4939-6403-1_1
8. Chapter 11. Rana B and Joshi GK. Electrophoresis: Basic principle, types and applications. Bhatt AK, Bhatia RK and Bhalla TC, ed. Basic Biotechniques for Bioprocess and Bioentrepeneurship. First edition. Academic Press; 2023:183-193. doi:10.1016/B978-0-12-816109-8.00011-8
9. Stolz A, Jooβ K, Höcker O, Römer J, Schlecht J and Neusüβ C. Recent advances in capillary electrophoresis-mass spectrometry: Instrumentation, methodology and applications. Electrophoresis. 2019; 40:79-112. doi:10.1002/elps.201800331
10. Chapter 16. Álvarez-Rivera G, Cifuentes A and Castro Puyana M. Electrophoretic technique: Capillary zone electrophoresis. Sun D-W, ed. Modern Techniques for Food Authentication. Second edition. Academic Press; 2018:659-685. doi:10.1016/B978-0-12-814264-6.00016-5
11. Zhu Z, Lu JJ and Liu S. Protein separation by capillary gel electrophoresis: A review. Anal Chim Acta. 2012; 709:21-31. doi:10.1016/j.aca.2011.10.022
12. Bhimwal R, Rustandi RR, Payne A and Dawod M. Recent advances in capillary gel electrophoresis for the analysis of proteins. J Cromatogr A. 2022; 1682:463453. doi:10.1016/j.chroma.2022.463453
13. Hajba L, Jeong S, Chung DS and Guttman A. Capillary gel electrophoresis of proteins: Historical overview and recent advances. Trends Analyt Chem. 2023; 162:117024. doi:10.1016/j.trac.2023.117024
14. Rodríguez-Díaz R, Wehr T and Zhu M. Capillary isoelectric focusing. Electrophoresis. 1997; 18:2134-2144. doi:10.1002/elps.1150181204
15. Kilár F. Recent applications of capillary isoelectric focusing. Electrophoresis. 2003; 24:3908-3916. doi:10.1002/elps.200305650
16. Silvertand LHH, Sastre Toraño J, van Bennekom WP and de Jong GJ. Recent developments in capillary isoelectric focusing. J Cromatogr A. 2008; 1204:157-170. doi:10.1016/j.chroma.2008.05.057
17. Gebauer P and Boček P. Recent progress in capillary isotachophoresis. Electrophoresis. 2002; 23:3858-3864. doi:10.1002/elps.200290006
18. Malá Z and Gebauer P. Recent progress in analytical capillary isotachophoresis (2018-March 2022). J Chromatogr A. 2022; 1677:463337. doi:10.1016/j.chroma.2022.463337
19. Tarabe S. Capillary separation: Micellar electrokinetic chromatography. Annu Rev Anal Chem. 2009; 2:99-120. doi:10.1146/annurev.anchem.1.031207.113005
20. Hancu G, Simon B, Rusu A, Mircia E and Gyéresi Á. Principles of micellar electrokinetic capillary chromatography applied in pharmaceutical analysis. Adv Pharm Bull. 2013; 3(1):1-8. doi:10.5681/apb.2013.001
21. Altria KD. Overview of capillary electrophoresis and capillary electrochromatography. J Chromatogr A. 1999; 856:443-463. doi:10.1016/S0021-9673(99)00830-4
22. Bartle KD and Myers P. Theory of capillary electrochromatography. J Chromatogr A. 2001; 916:3-23. doi:10.1016/S0021-9673(01)00709-9
23. Eeltink S, Rozing GP and Kok WT. Recent applications in capillary electrochromatography. Electrophoresis. 2003; 24:3935-3961. doi:10.1002/elps.200305638
24. D’Orazio G, Asensio-Ramos M, Fanali C, Hernández-Borges J and Fanali S. Capillary electrochromatography in food analysis. Trends Analyt Chem. 2016; 82:250-267. doi:10.1016/j.trac.2016.06.012
25. Weston A and Brwon PR. HPLC and CE. Principles and Practice. Academic Press. First edition; 1997:1-280. ISBN: 0-12-136640-5
26. François I, Sandra K and Sandra P. Comprehensive liquid chromatography: Fundamental aspects and practical considerations – A review. Anal Chim Acta. 2009; 641:14-31. doi:10.1016/j.aca.2009.03.041
27. Righetti G, Gelfi C and D’Acunto MR. Recent progress in DNA analysis by capillary electrophoresis. Electrophoresis. 2002; 23:1361-1374. doi:10.1002/1522-2683(200205)23:10%3C1361::AID-ELPS1361%3E3.0.CO;2-J
28. Durney BC, Crihfield CL and Holland LA. Capillary electrophoresis applied to DNA: Determining and harnessing sequence and structure to advance bioanalyses (2009-2014). Anal Bioanal Chem. 2015; 407:6923-6938. doi:10.1007/s00216-015-8703-5
29. Dovichi NJ and Zhang J. How capillary electrophoresis sequenced the human genome. Angew Chem Int Ed. 2000; 39(24):4463-4468. doi:10.1002/1521-3773(20001215)39:24%3C4463::aid-anie4463%3E3.0.co;2-8
30. Wan Y, Kertesz M, Spitale RC, Segal E and Chang HY. Understanding the transcriptome through RNA structure. Nat Rev Genet. 2011; 12:641-655. doi:10.1038/nrg3049
31. Murakami Y, Kubo S, Tamori A, Itami S, Kawamura E, Iwaisako K et al. Comprehensive analysis of transcriptome and metabolome analysis in intrahepatic cholangiocarcinoma and hepatocellular carcinoma. Sci Rep. 2015; 5:16294. doi:10.1038/srep16294
32. Perovic J, Silvar C, Koenig J, Stein N, Perovic D and Ordon F. A versatile fluorescence-based multiplexing assay for CAPS genotyping on capillary electrophoresis systems. Mol Breeding. 2013; 32:61-69. doi:10.1007/s11032-013-9852-x
33. Minucci A, Canu G, De Bonis M, Delibato E and Capoluongo E. Is capillary electrophoresis on microchip devices able to genotype uridine diphosphate glucuronosyltransferase 1A1 TATA-box polymorphisms? J Sep Sci. 2014; 37(12):1521-1523. doi:10.1002/jssc.201400235
34. Vallejo-Cordoba B and González-Córdova A. Capillary electrophoresis for the analysis of contaminants in emerging food safety issues and food traceability. Electrophoresis. 2010; 31:2154-2164. doi:10.1002/elps.200900777
35. Vergara-Barberán M, Simó-Alfonso EF, Herrero-Martínez JM and Benavente F. Accurate determination of the milk protein allergen β-lactoglobulin by on-line aptamer affinity solid-phase extraction capillary electrophoresis-mass spectrometry. Talanta. 2023; 259:124542. doi:10.1016/j.talanta.2023.124542
36. Toutotunji MR, Van Leeuwen MP, Oliver JD, Shrestha AK, Castignolles P and Gaborieau M. Quantification of sugars in breakfast cereals using capillary electrophoresis. Carbohydr Res. 2015; 408:134-141. doi:10.1016/j.carres.2015.03.008
37. Hernández A, Martín A, Aranda E, Bartolomé T and Córdoba MdG. Detection of smoked paprika “Pimentón de la Vera” adulteration by free zone capillary electrophoresis (FZCE). J Agric Food Chem. 2006; 54:4141-4147. doi:10.1021/jf060349r
38. Brown RS, Luong JHT, Szolar OHJ, Halasz A and Hawari J. Cyclodextrin-modified capillary electrophoresis: Determination of polycyclic aromatic hydrocarbons in contaminated soils. Anal Chem. 1996; 68(2):287-292. doi:10.1021/ac950801y
39. Sovocool GW, Brumley WC and Donnelly JR. Capillary electrophoresis and capillary electrochromatography of organic pollutants. Electrophoresis. 1999; 20(15-16):3297-3310. doi:10.1002/(SICI)1522-2683(19991001)20:15/16%3C3297::AID-ELPS3297%3E3.0.CO;2-9
40. Ali I and Aboul-Enein HY. Determination of metal ions in water, soil and sediment by capillary electrophoresis. Anal Lett. 2002; 35(13):2053-2076. doi:10.1081/AL-120015519
41. Dinelli G, Vicari A and Catizone P. Monitoring of herbicide pollution in water by capillary electrophoresis. J Chromatogr A. 1996; 733(1-29):337-347. doi:10.1016/0021-9673(95)00954-X
42. Ahrer W, Scherwenk E and Buchberger W. Determination of drug residues in water by the combination of liquid chromatography or capillary electrophoresis with electrospray mass spectrometry. J Chromatogr A. 2001; 910(1):69-78. doi:10.1016/S0021-9673(00)01187-0
43. Martínková E, Křížek T and Coufal P. Determination of nitrites and nitrates in drinking water using capillary electrophoresis. Chem Pap. 2014; 68(8):1008-1014. doi:10.2478/s11696-014-0548-4
44. Fuiko R, Saracevic E, Koenka IJ, Hauser PC and Krampe J. Capillary electrophoresis for continuous nitrogen quantification in wastewater treatment processes. Talanta. 2019; 195:366-371. doi:10.1016/j.talanta.2018.11.056
45. Suntorksuk L. Recent advances of capillary electrophoresis in pharmaceutical analysis. Anal Bioanal Chem. 2010; 398:29-52. doi:10.1007/s00216-010-3741-5
46. Shah M, Patel N, Tripathi N and Vyas VK. Capillary electrophoresis methods for impurity profiling of drugs: A review of the past decade. J Pharm Anal. 2022; 12(1):15-28. doi:10.1016/j.jpha.2021.06.009
47. Haginaka J. Enantiomer separation of drugs by capillary electrophoresis using proteins as chiral selectors. J Chormatogr A. 2000; 875(1-2):235-254. doi:10.1016/S0021-9673(99)01168-1
48. Koppenhoefer B, Zhu X, Jakob A, Wuerthner S and Lin B. Separation of drug enantiomers by capillary electrophoresis in the presence of neutral cyclodextrins. J Chromatogr A. 2000; 875(1-2):135-161. doi:10.1016/S0021-9673(99)01210-8
49. Weissinger EM, Wittke S, Kaiser T, Haller H, Bartel S, Krebs R et al. Proteomic patterns established with capillary electrophoresis and mass spectrometry for diagnostic purposes. Kidney Int. 65(6):2426-2434. doi:10.1111/j.1523-1755.2004.00659.x
50. Mischak H, Coon JJ, Novak J, Weissinger EM, Schanstra JP and Dominiczak AF. Capillary electrophoresis-mass spectrometry as a powerful tool in biomarker discovery and clinical diagnosis: An update of recent developments. Mass Spectrom Rev. 2009; 28:703-724. doi:10.1002/mas.20205
51. Yang Z and Sweedler JV. Application of capillary electrophoresis for the early diagnosis of cancer. Anal Bioanal Chem. 2014; 406:4013-4031. doi:10.1007/s00216-014-7722-y
52. Moser AC and Hage DS. Capillary electrophoresis-based immunoassays: Principles and quantitative applications. Electrophoresis. 29:3279-3295. doi:10.1002/elps.200700871
53. Moser AC, Willicott CW and Hage DS. Clinical applications of capillary electrophoresis-based immunoassays. Electrophoresis. 2014; 35:937-955. doi:10.1002/elps.201300421
54. Huang C-C, Cao Z, Chang H-T and Weihong T. Protein-protein interaction studies based on molecular aptamers by affinity capillary electrophoresis. Anal Chem. 2004; 76(23):6973-6981. doi:10.1021/ac049158i
55. He X, Ding Y, Li D and Lin B. Recent advances in the study of biomolecular interactions by capillary electrophoresis. Electrophoresis. 2004; 25:697-711. doi:10.1002/elps.200305727
56. Faserl K, Sarg B, Gruber P and Lindner HH. Investigating capillary electrophoresis-mass spectrometry for the analysis of common post-translational modifications. Electrophoresis. 2018; 39:1208-1215. doi:10.1002/elps.201700437
57. Gomes FP and Yates III JR. Recent trends of capillary electrophoresis-mass spectrometry in proetomics research. Mass Spectrom Rev. 2019; 38:445-460. doi:10.1002/mas.21599
58. Cruces-Blanco C, Gámiz-García L and García-Campaña AM. Applications of capillary electrophoresis in forensic analytical chemistry. Trend Analyt Chem. 2007; 26(3):215-226. doi:10.1016/j.trac.2006.12.007
59. Mayes C, Seashols-Williams S and Hughes-Stamm S. A capillary electrophoresis method for identifying forensically relevant body fluids using miRNAs. Leg Med. 2018; 30:1-4. doi:10.1016/j.legalmed.2017.10.013
60. Bertaso A, De Palo EF, Cirielli V and Tagliaro F. Lactate determination in human vitreous humour by capillary electrophoresis and time of death investigation. Electrophoresis. 2020; 41:1039-1044. doi:10.1002/elps.201900462
61. Strege MA and Lagu AL. Capillary electrophoresis as a tool for the analysis of protein folding. J Chromatogr A. 1993; 652(1):179-188. doi:10.1016/0021-9673(93)80658-U
62. Guttman A. Capillary electrophoresis in the N-glycosylation analysis of biopharmaceuticals. Trends Analyt Chem. 2013; 48:132-143. doi:10.1016/j.trac.2013.04.006
63. Szigeti M and Guttman A. Automated N-glycosylation sequencing of biopharmaceuticals by capillary electrophoresis. Sci Rep. 2017; 7:11663. doi:10.1038/s41598-017-11493-6