Iron Oxide May Explain Mysterious Anomalies Under the Earth’s Surface
Iron oxide’s melting point is much higher than previously thought, lending credence to a theory that explains unusual behavior near the planet’s core.
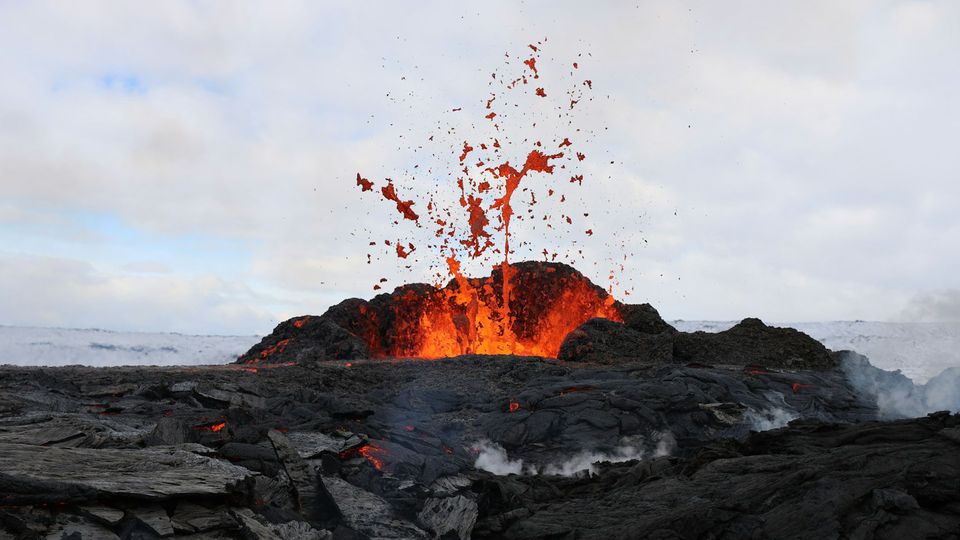
Complete the form below to unlock access to ALL audio articles.
Under the Earth’s surface, just above the planet’s iron metal core, lies the core–mantle boundary (CMB). This extreme region separates the planet’s outer core from the thick layer of molten rock mantle and lies approximately 1,800 miles beneath our feet.
Despite seeming a long way down, the CMB does have an impact on our world above. Over the course of tens of millions of years, plumes of material from the CMB can ascend upwards through the center of the Earth,shaping the volcanic activity, chemistry and plate tectonics that we experience at the surface.
By measuring seismic waves, scientists have uncovered the existence of several strange regions in and around the CMB, where the waves travel much more slowly than expected. For some time, scientists have speculated that these regions could be particularly rich in iron oxide, which would explain the wave slowing. But could iron oxide solids even exist at such extreme temperatures and pressures? According to a new study published in Nature Communications, it seems likely.
Does an ancient protoplanet lie under the Earth’s surface?
In early November, geophysicists published a paper in Nature suggesting two strange “blobs” of unusual material found in the Earth’s lowermost mantle could be the remnants of a hypothesized protoplanet that once collided with a young Earth. The paper, which focuses on two large low-velocity provinces (LLVPs) located deep underneath the Pacific Ocean and African continent, suggests that after colliding with the Earth, fragments of the iron-rich protoplanet could have become trapped under the Earth’s surface and formed these LLVPs.
In addition to these LLVPs, there are two major ultra low velocity zones (ULVZs) that can be found on the CMB, one slightly to the west of Hawai’i and the other near Samoa. Like the LLVPs, scientists hypothesize that the slow pace of seismic waves through ULVZs is due to high levels of iron oxides present in these areas. But very little is known about these ULVZs.
"Because we can't simply go down to the CMB and take measurements, there are many open questions about a region that is so important to our planet's evolution," said Jennifer Jackson, the William E. Leonhard Professor of Mineral Physics at the California Institute of Technology (Caltech) and the senior author of the new Nature Communications study. "Why do the ULVZs exist and what are they made of? What do they teach us about how the Earth evolved and what role the region plays in the dynamics of the Earth? Are the blobs solid or molten at the extreme conditions at the CMB?"
Curious about whether iron oxides could exist in a solid form in such extreme conditions, Jackson’s lab conducted detailed measurements on the behavior of iron oxide under a range of temperatures and pressures similar to those found in the CMB. From this, the researchers were able to create a phase diagram that proves that iron oxide can remain solid even at very high temperatures.
This represents the strongest evidence to date that solid iron-rich regions could be a realistic explanation for the strange behavior seen in ULVZs. Such iron-rich regions may even play a pivotal role in explaining volcanic activity, such as deep-seated mantle plume generation.
Novel spectroscopy method examines iron under harsh conditions
For their study, Jackson’s team made use of a novel spectroscopic technique that had been developed by the team, to observe the dynamic configuration of atoms in very short timeframes.
The technique, called Mössbauer spectroscopy, can take samples smaller than the average width of a human hair and detect the precise temperature at which the material begins to transition from a solid to a liquid, based on the movement of atoms in the sample as they begin to fall out of their rigidly repeating solid structures and become more fluid.
"We use Mössbauer to answer questions about the dynamic movement of iron atoms," said former Caltech graduate student Vasilije Dobrosavljevic, the study’s first author. "Over a short timeframe of around 100 nanoseconds, we want to know: do they barely move, as in a solid, or do they move a lot, as in a liquid? Our new study complements Mössbauer spectroscopy with an independent method, X-ray diffraction, that lets us observe the positions of all atoms in the sample."
After dozens of experiments, Dobrosavljevic, Jackson and collegues discovered that iron oxide melts at over 4,000 Kelvins (approximately 6,700 degrees Fahrenheit) when at pressures equal to those found in the CMB. This is higher than previously estimated but also, crucially, is higher than the recent temperature estimates for the present-day CMB, meaning that solid iron oxides are a real possibility in explaining the composition of ULVZs.
An unexpected discovery
The study also delivered another, more unexpected, result. At atmospheric pressure, iron oxide samples do have atomic-scale defects in them. For every 100 oxygen atoms, there are only around 95 iron atoms in the same space, meaning that 5 iron atoms are “missing”. Materials scientists are interested in such things as these defects can have a significant impact on the properties of materials at a larger scale. For example, such defects could affect how a substance deforms under pressure or how it conducts heat.
Such defects are also interesting to geophysicists, who can use defect information to understand planetary interiors by studying where heat flow and material deformation drive various planetary dynamics. The behavior of such defects at the kinds of pressures and temperatures seen in the CMB were unknown until now.
With their new methodology, Dobrosavljevic and his team were able to observe how, at temperatures several hundred Kelvins lower than iron oxide’s melting point, the minor atomic defects in the material can begin to shift around and become “disordered” within the sample.
The researchers believe this could explain the previous lower estimates for iron oxide’s melting point – rather than detecting the melting of the whole crystal structure, those experiments were seeing defect shifts.
"Before the solid crystal transitions to a liquid, we see that the defect structure undergoes a transition from ordered to disordered," Dobrosavljevic explained. "Now we want to know what effect this newly discovered transition has on the physical properties of iron-rich regions like the ULVZ? How do the defects affect the transport of heat, and what does it mean for the formation and generation of upwelling plumes that reach the surface? These questions will guide further research."
Reference: Dobrosavljevic VV, Zhang D, Sturhahn W, et al. Melting and defect transitions in FeO up to pressures of Earth’s core-mantle boundary. Nat Commun. 2023;14(1):7336. doi: 10.1038/s41467-023-43154-w
This article is a rework of a press release issued by the California Institute of Technology. Material has been edited for length and content.