Next-Gen mRNA Technology: A Crucial Pandemic Preparedness Tool
Explore why continued investment in this promising field is crucial for maintaining our pandemic preparedness.
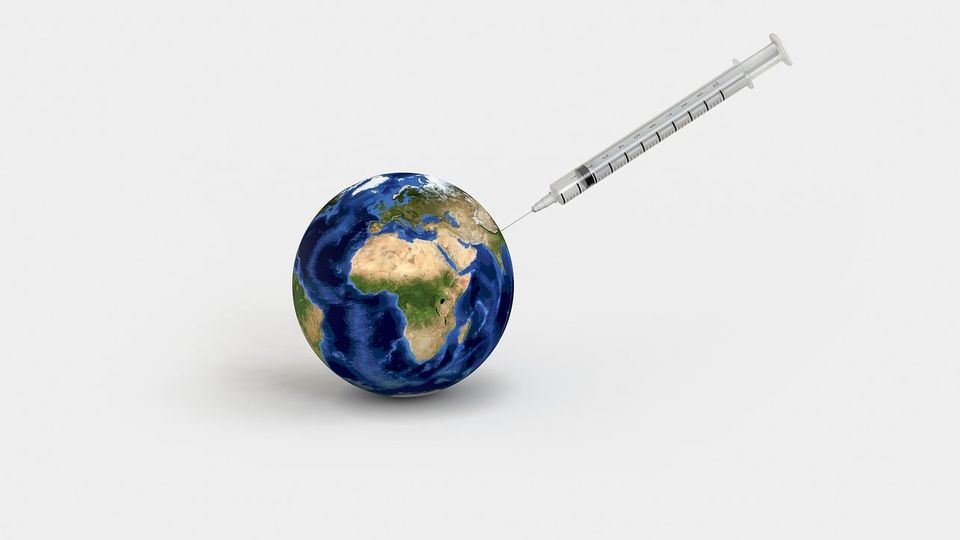
Complete the form below to unlock access to ALL audio articles.
Since its discovery, scientists have worked to harness the power of messenger RNA (mRNA) to treat and prevent diseases. These research efforts laid the foundation for the rapid development of vaccines to control the spread of SARS-CoV-2. While the height of the pandemic has passed, there is a broad consensus among the scientific community that the potential for future epidemics and pandemics remains high. Nevertheless, it is far from inevitable that the next disease outbreak will be as devastating as the COVID-19 pandemic. We continue to improve our ability to combat and control these diseases, and next-gen mRNA technology is one of the most valuable tools in our arsenal. Continued investment in this promising field is crucial for maintaining our pandemic preparedness.
Prior investments in mRNA research paid dividends during the COVID-19 pandemic
The genetic sequence of SARS-CoV-2 was published on January 12, 2020, and a mere three months later, the first human subject was dosed with the Pfizer-BioNTech vaccine in a Phase I clinical trial. Decades of research provided the crucial foundation for the rapid deployment of mRNA vaccines to control the COVID-19 pandemic.
There are several key elements of a successful mRNA vaccine. First, the delivery vehicle must successfully transport the ribonucleic sequence to the appropriate tissues and cellular compartments with minimal immunogenicity. Lipid nanoparticles (LNPs) were used with great success in vaccines against COVID-19. The LNP vesicle used for the Pfizer vaccine was a variation of the LNPs already tested in other clinical trials, such as those for CMV and hMPV-PIV3 vaccines. This precedent reduced the regulatory burden for COVID-19 vaccines and provided evidence of the LNP’s safety for use in clinical settings.
Similarly crucial for mRNA therapeutics is the appropriate chemical modification of the mRNA, such as the addition of a methylated 5’ cap. Characteristics of the 5’ cap dramatically impact translational efficiency, mRNA stability and immunogenicity. Recent improvements to artificially generated 5’ caps allow smaller dosages of mRNA to exert greater effects, increasing the tolerability of mRNA treatments in patients. Other advancements in mRNA production have improved the removal of double-stranded RNA (dsRNA) and other contaminants, yielding higher-purity products. These refinements in mRNA production were pivotal in ensuring that COVID-19 vaccines were safe and efficacious in the general population.
mRNA readily lends itself to large-scale vaccine production
Manufacturing of mRNA vaccines is a more streamlined process compared to traditional vaccines. Unlike other vaccines, mRNA vaccines are nucleotide sequence-based and don’t require the production of a specific protein or culturing of a dangerous pathogen. The benefits of this are twofold. First, there is uniformity in the raw material and facility requirements, regardless of the pathogen. This makes prediction of supply demands easier and allows governments to invest proactively to reserve the necessary reagents. Second, facilities capable of containing highly infectious pathogens are no longer required, reducing biosafety demands that can complicate production and staffing.
Next-gen mRNA technology also benefits from being generated in a cell-free system. Vaccines against influenza, yellow fever and other viruses are grown in chicken eggs, which creates a dependency on egg production when scaling up vaccine assembly. Generating vaccines in a cell-free system also significantly reduces the risk of bacterial contamination. Together, these factors significantly reduce production costs and expedite timelines, making mRNA vaccines an economically preferable choice.
In addition, mRNA vaccines are inherently flexible and easily altered in response to rapidly mutating pathogens. As a nucleotide sequence-based technology, the vaccine’s efficacy can be improved without altering the biological properties of the mRNA strand or the delivery vehicle. This allows for efficient, real-time updating of vaccines alongside the elimination of safety concerns associated with the introduction of novel reagents to a vaccine formulation. The flexibility of mRNA technology enabled the rapid release of updated COVID-19 vaccines that could more effectively target novel circulating strains. This will undoubtedly prove useful in combatting future pandemics.
Continued investment in mRNA technology will have both short- and long-term benefits
Despite the success of mRNA vaccine technology during the COVID-19 pandemic, further improvements will be necessary for the field to reach its full potential. For instance, supply chain issues create bottlenecks in vaccine production, making it difficult to generate sufficient supply for a global vaccination campaign. Global stakeholders must work to develop infrastructure and form the international relationships that are required for a streamlined supply chain. Additionally, it is essential that appropriate standards are established for the involved reagents so that quality mRNA vaccines can confidently be produced at scale.
Researchers are also actively pursuing several exciting scientific advancements in mRNA technology. One significant area of interest is in fine-tuning the LNP delivery system. Modifying the lipids used for packaging mRNA could improve the vaccine's potency and increase tolerability. Additional research aimed at improving the stability of LNPs could also increase tolerance to temperature fluctuations during transport. This is especially important in regions with less established infrastructure for maintaining frozen samples. Finally, we continue to learn more about selecting mRNA characteristics that enhance treatments. This includes modification of the 5’ cap for increased translation, alteration of incorporated nucleotides to avoid activation of the immune system and exploration of self-replicating mRNA treatments through packaging with an RNA-dependent RNA polymerase.
The potential applications of mRNA therapeutics extend far beyond viral vaccinations. This technology holds great promise for developing therapeutic cancer vaccinations, which prime the immune system to fight cancer cells effectively. There are also exciting opportunities for next-gen mRNA technology in the development of protein-replacement therapies that could treat various genetic diseases. By continuing to invest in next-gen mRNA technology, we can drive medical advances today while protecting ourselves from the potential pandemics of tomorrow.
About the author:
Drew Burch is executive vice president of nucleic acid products at TriLink Biotechnologies.